Our research covers a variety of topics related to the dynamics of plate tectonics and mantle convection in subduction zones – where two tectonic plates converge causing one plate to sink into the mantle. We use finite element computer simulations for most of our research. The software we use is developed and maintained by the research community and the Computational Infrastructure for Geodynamics (CIG). This research is divided into four sub-topic areas and some other projects:
- Time-Dependent Slab Dynamics
- Outer-rise Strength and Faulting
- Upper Mantle Viscosity Structure
- Interactive 3D Visualization and Immersive Environments
- Other Topics (Osbourn Trough, Transition Zone, Discontinuous Galerkin,…)
Time-Dependent Slab Dynamics
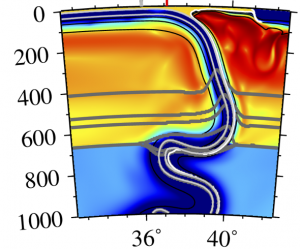
Snap-shot from 2D time-dependent model of subduction. Phase transitions cause the slab to buckle and fold and the trench location moves in response to slab deformation. An arc rifting event has caused extension of the overriding plate.
Deformation of sinking tectonics plates (slabs) depends on the strength of the slab, the mantle viscosity structure, and the evolving density structure. Determining how slabs drive plate motions is important for understanding why we have plate tectonics on Earth and what are the necessary conditions for plate tectonics on other planets. Over many years and projects we have systematically increased the complexity and realism of modeled processes and parameters to investigate how the balance of driving and resisting forces leads to the observed shape of slabs, and motions of plates and plate boundaries. For example we have shown that the non-linear rheology of the upper mantle allows slabs to deform less, but realistic phase change structure cause slab buckling and folding. Slab strength also determines when and how a slab breaks-off from the surface plate as a spreading ridge approaches the subduction zone. However, slab deformation also depends on overriding plate thickness and 3D flow near the edges of slabs. Our studies of time-dependent slab dynamics use 2D or 3D simulations that are designed so that the results can either be compared to observations from a specific location (e.g., Baja California) or to systematic analysis of global observations.
- Episodic Slab Dynamics and Trench Motion: Effect of Phase Transitions & Upper Plate (Pubs: 39, 41)
- Spontaneous Arc Rifting (Pubs: 43)
- Overriding Plate Structure: Slab Dip and Plate Motion (Pubs: 26, 35, 37, 40)
- Ridge-Trench Interaction and Slab Break-off (Pubs: 17, 18, 19)
- Non-linear Rheology and Slab Dynamics (Pubs: 7, 10, 12, 16)
Outer-Rise Strength and Faulting
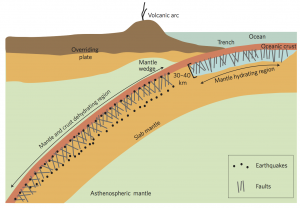
Sketch of subducting plate faults and region of weakening in the bending region. Shallow and intermediate depth faults may be related to hydration and dehydration of the slab along outer-rise faults.
About 50-100 km seaward of the trench the subducting plate starts to show signs of bending as its sinks into the mantle. This region, called the outer-rise, is characterized by large normal faults that extend into the mantle portion of the subducting plate (> 10-20 km deep). Outer-rise faulting is important to understand because these faults are thought to be responsible for hydrating the subducting plate at the surface and then releasing water to the mantle as the slab heats up. Also, because the plate is cold at the Earth’s surface it is quite strong and it needs to weaken in order to bend into the mantle. By looking at the relationship between the observed topography and gravity signal we founds that this weakening is dramatic and happens over distances of less than 50 km. Numerical simulations also show that the creation of faults (spacing and depth extent) depends on plate age, but is not very sensitive to the frictional properties of the rock.
- Rheologic Constraints on Outer-rise Faulting (Pubs: 34)
- Plate Strength During Bending: Gravity-Topography Admittance (Pubs: 8, 29)
- Frictional strength of outer-rise faults (Pubs: 11)
Upper Mantle Viscosity Structure
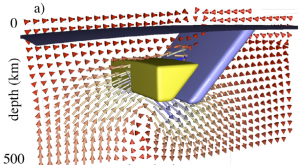
View of 3D low viscosity wedge and slab with slice of mantle flow shown arrows (colored by speed) from Billen and Jadamec, G-cubed, 2012.
Over millions of years the slow deformation of the Earth’s mantle occurs through solid-state viscous flow – that is, the mantle is a solid (it is NOT melted) but it deforms like a fluid. Therefore, the viscosity structure of the slab and mantle have a first-order control on how plates and the mantle move and deform. Observations of deformation of the Earth’s surface (uplift and subsidence) caused by internal deformation of the mantle, as well of the speed an direction of plate motions can provide important constraints on the viscosity structure. For these kinds of studies we typically use instantaneous flow models in which we defined the viscosity structure (which can depend on temperature, compositions, stress,…) and the driving density variations (extra weight of the slab) and determine the flow and deformation for a single instant in time (usually the present day). Using this approach we have found that the mantle wedge (between the slab and the overriding plate) is very weak due to the stress dependence of mantle viscosity. This low viscosity can lead to locally rapid flow (greater than 20 – 40 cm/yr) compared to the typically slower motion of tectonic plate (5-10 cm/yr). However, we have also shown that observations of dynamic topography and geoid can be “tricked” by internal weakening of the slab and the strong variation in strength between the slab and surrounding mantle. In this case, more than one viscosity structure can match the observations, and therefore other approaches (time-dependent simulations) and observations (slab shape, plate motion) are needed to constrain the models.
- Low Viscosity Wedge & Fast Flow (Pubs: 30)
- Southern Alaska: 3D Mantle Flow and Surface Deformation ( Pubs: 21, 25, 27, 32)
- Dynamic Topography & Geoid Constraints (Pubs: 2, 4, 5, 28)
3D Visualization and Immersive Environments
Geological and geophysical data are inherently three-dimensional (3D), but for a long time we have struggled with how to visualize, analyze and understand this data on a flat piece of paper or computer screen. This struggle takes time and energy away from the real scientific pursuit. Through long-term participation in in the KeckCAVES, we have had the opportunity to use sophisticated 3D visualization tools and immersive environments in our research and for teaching. These projects have aimed to both demonstrate the utility of interactive and immersive environments for research and teaching, and to use these tools for our own research. We have used these tools to design the 3D structure of slabs used in simulations and to analyze the flow from these simulations (see publications and PhD thesis by Margarete Jadamec and Erin Burkett), and to explore and analyze deep seismicity with an aim to understanding the internal deformation of slabs in the transition zone (see MS thesis by John Bikoba). This research has also lead to the development of tools for teaching students how to visualize complex 3D geologic structures.
- Interactive and Immersive Environments (Pubs: 9, 13, 14)
- 3D Visualization of Geological & Geophysical Data (Pubs: 22, 23, 24, 31)
Other Topics
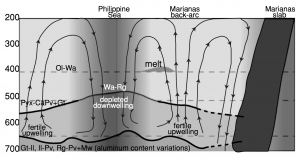
Interpretation of seismic reflections in the mantle near the Mariana slab from Thomas and Billen, Geophysical Journal International, 2009.
Over the years our group has also pursued a range of other topics related to our other primary research areas. These projects have focused on other geodynamical topics such as using seismological observations to better constrain the compositional variation in the transition zone, or determining the role of lithospheric instabilities in causing surface deformation in the Sierra Mountains of California. We have also worked with applied mathematicians to improve methods used in numerical simulations. In particular we collaborated on the development of a Discontinuous Galerkin method for advection of composition fields in viscous flow models.
- Transition Zone Structure (Pubs: 16, 20)
- Lithospheric Instabilities (Pubs: 6, 36)
- The Osbourn Trough (Pubs: 1)
- Mathematical Methods (Pubs: 31, 42)